Introduction
Organs-on-chips are a new class of microengineered laboratory models that combine several of the advantages of current in vivo and in vitro models. In this review, the advances that have been made in the development of organ-on-chip models of the blood-brain barrier (BBBs-on-chips) and the challenges that are still ahead are summarized.
Blood-brain barrier structure and function
The blood-brain barrier (BBB) comprises specialized endothelial cells and separates blood from brain interstitial fluids. Together with the choroid plexus which forms the blood-cerebrospinal fluid barrier, and the arachnoid epithelium, this barrier partitions blood and neural tissues in order to provide vital homeostasis in the brain. The BBB serves as a physical and functional barrier which regulates passive and active transport, as well as a metabolic and immunological barrier. The physical barrier is formed by the endothelial cells that are linked by tight junction proteins such as zonula occludens 1 (ZO-1) and claudin. These proteins form complexes that limit permeation of ions and hydrophilic agents via paracellular pathways. The active transport barrier results from the expression of specific membrane transporters and vesicular mechanisms for exchange of specific essential nutrients and waste, and multidrug resistance transporters such as P-glycoprotein (Pgp) that regulate efflux of potentially harmful agents, including lipophilic agents. The metabolic barrier is formed by enzymes that metabolize toxic compounds both intracellularly and extracellularly. As a result of the physical and metabolic barrier, 98% of small-molecule and 100% of large-molecule drugs cannot cross the BBB. Finally, the immunological barrier results from specialized regulation of the recruitment and transport of leukocytes and innate immune elements by the endothelium.
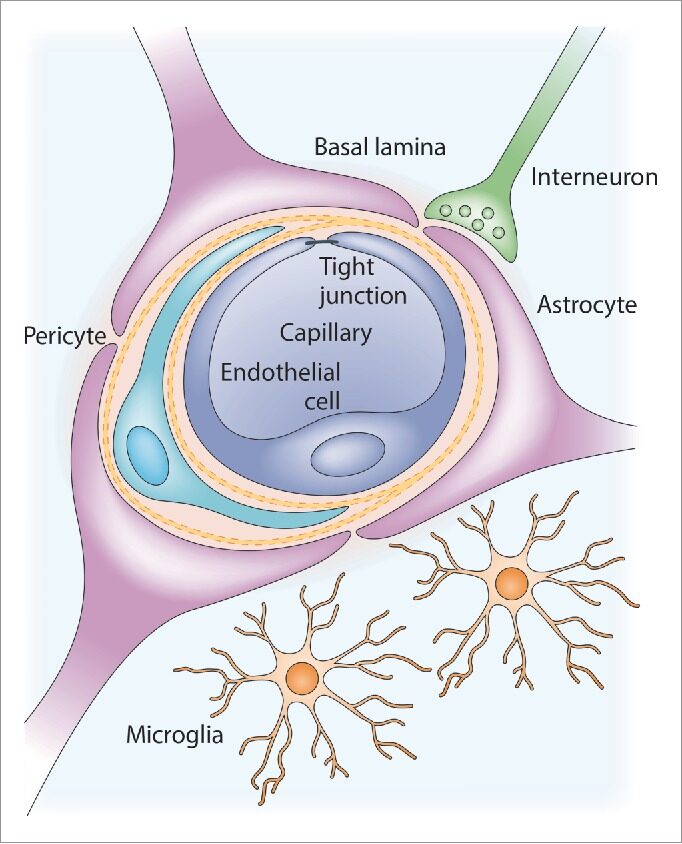
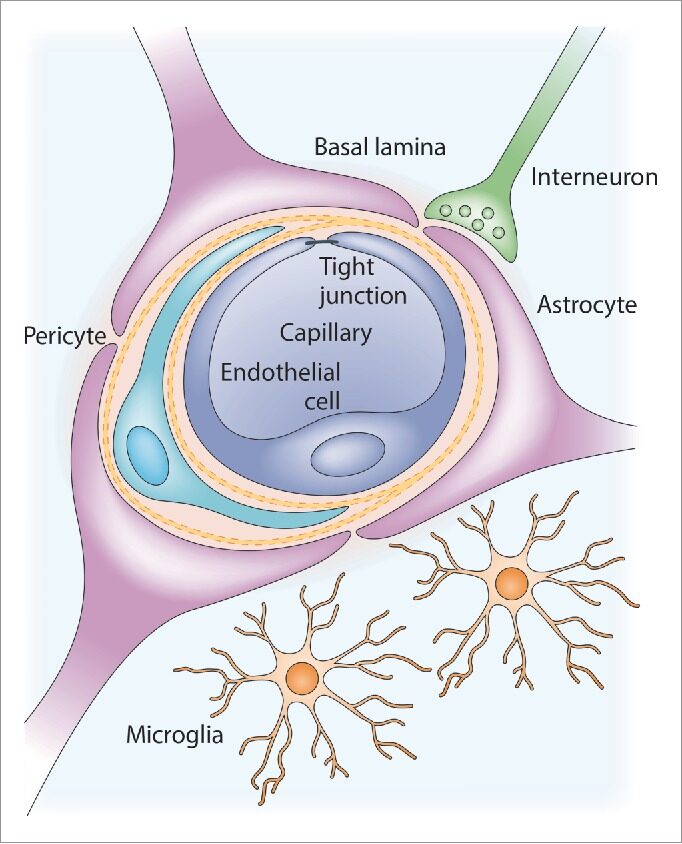
Figure 1.Anatomy of the neurovascular unit. A brain capillary comprised of specialized brain endothelial cells forms the blood-brain barrier (BBB). This capillary is surrounded by basal lamina (basement membrane), pericytes and astrocytic end-feet. Also, microglia and neurons are in close contact with the BBB. Adapted from source
Current in vitro and in vivo models
In vivo techniques have provided the most reliable information in BBB research and are still regarded as the gold standard. In the pharmaceutical industry drug candidates are normally tested in animals before they are tested in humans. In these models the effects of drugs or treatments at the cellular, tissue, organ and systemic level can be monitored. Moreover, animal models allow the study of pharmacodynamics and pharmacokinetics, as well as of immunological responses. A general advantage of animal models is that they can represent the complexity of the BBB environment and individual diversity found in humans. However, in vivo animal studies are costly, labor-intensive and ethically contentious. In addition, the translation of animal models to the human clinic is difficult, evidenced by the statement that more than 80% of candidate drugs that were successfully tested in animal models failed in clinical trials. This is partly caused by poor methodology and regulation of (some) animal experiments,10-13 but also by inadequate reproduction of human pathophysiology by (genetically modified) animals10-12 and by species-to-species variations in expression profiles of e.g., transporter proteins.
Organs-on-chips
To combine the advantages of in vivo and current in vitro models of tissues and organs, a new class of in vitro models has recently been introduced: organs-on-chips. These so-called chips are microfluidic devices in which tissues can be cultured in an environment that is engineered in such a way that it better replicates the in vivo microenvironment of that tissue. This more physiologically relevant microenvironment can be achieved by engineering geometrical, mechanical and biochemical factors from the in vivo environment into a microfluidic device. Another advantage of these organ-on-chip platforms is that imaging systems and sensors with real-time readouts can also be integrated. Like in conventional in vitro methods, human cells or tissues can be included in organs-on-chips. Furthermore, these devices can be used for personalized (or precision) medicine when cells from a specific donor or group of donors are used. Both healthy and diseased tissues can be mimicked and tested in the same controlled environment. Moreover, organs-on-chips promise to replicate organ-level functions and allow the study of pathophysiology on a higher level than could be achieved by conventional in vitro models. The comparison of organs-on-chips with current in vivo and in vitro methods is summarized in Table 1.
Table 1. Comparison of organs-on-chips to current in vivo and in vitro methods. Adapted from source
In vivo | In vitro | Organs-on-chips | |
Human tissue | No | Yes | Yes |
Personalized/precision medicine | No | Yes | Yes |
Realistic microenvironment | Yes | No | Yes |
Control over microenvironment | No | Yes | Yes |
Organ-level function | Yes | Limited | Potentially |
Real-time readouts | No | Limited | Yes |
High-throughput, parallelized testing | No | Yes | Possibly |
Pharmacodynamics / -kinetics | Yes | No | Potentially |
BBB-on-chip models
As shown in the previous paragraphs, the use of microfluidic in vitro BBB models can improve BBB modeling by having more realistic dimensions and geometries, and by exposing the endothelium to physiological fluid flow. In addition, in “BBBs-on-chips” not only the expression of specific markers can be tested (e.g., adherens and tight junction proteins), which can provide information on an organ-level function, but one can immediately study functionality: the permeability of the cell barrier. Permeability is now already routinely measured in compartmentalized cultures (e.g., Transwell models), but BBBs-on-chips hold promise to measure more BBB functions directly by incorporating sensors and real-time readouts. An additional example of a BBB function, which cannot be studied in Transwell, is the complex and specialized mechanism of recruitment of leukocytes at the BBB, analogous to the extravasation of leukocytes in the lung-on-a-chip in case of bacterial infection. In addition, the recently discovered glymphatic pathway, which clears solutes from the brain and probably plays a role in neurodegenerative diseases, can be studied for the first time using microfluidic devices in which physiologically relevant blood pressure, intracranial pressure and flows can be applied. When BBBs-on-chips are used to study such a complex biological phenomenon, they will provide deeper understanding of the BBB physiology and answer research questions that could not be answered before. BBBs-on-chips then provide an extra tool for the BBB researchers’ toolbox, next to classic in vitro cultures and in vivo animal studies. Depending on the research question, the most appropriate model can be chosen.
Current BBBs-on-chips
According to the information available, to this date, only ten publications of BBBs or NVUs-on-chips exist. In Figure 2, representative images are shown.
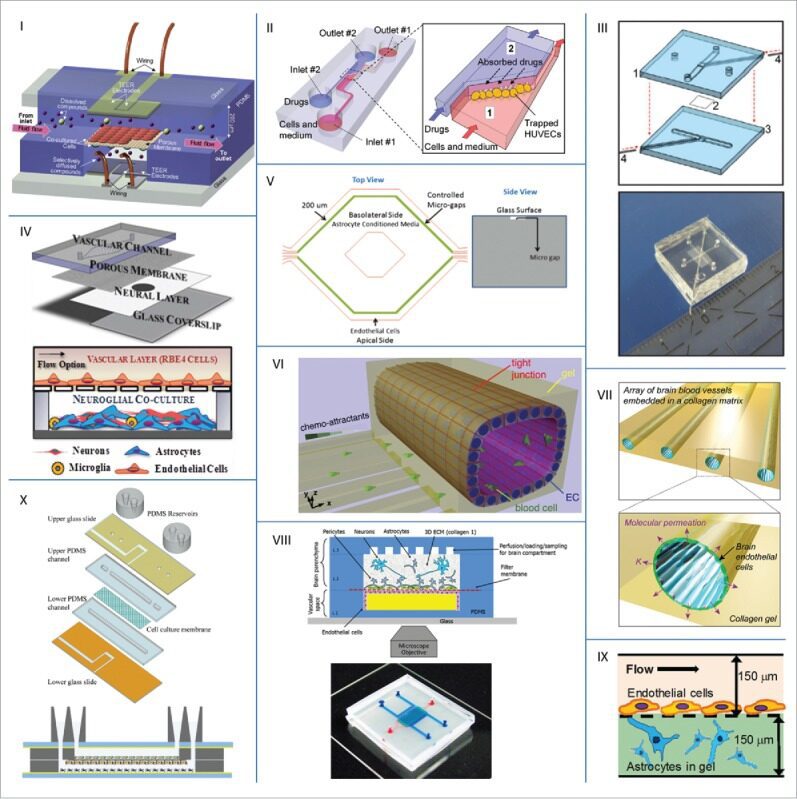
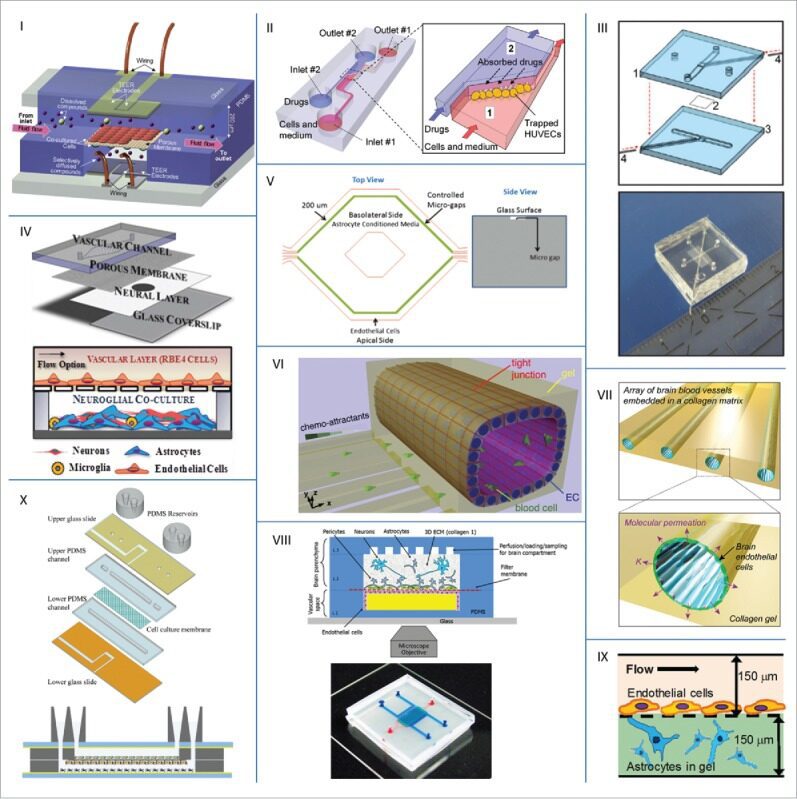
Figure 2. Examples of microfluidic BBB models from literature. Adapted from source
Standardization challenges
As evidenced by the body of literature summarized in the previous section, significant steps have been taken toward developing physiological BBBs-on-chips. These recently reported chips show promising improvements when compared to conventional Transwell models: the exposure to fluid flow resulted in better barrier function. and dynamic drug permeability studies in chips were found to be more predictive than in conventional static models.
Permeability
The key function of the BBB is to provide homeostasis in the brain, and more specifically to protect the brain from harmful substances in the blood. The performance of BBBs-on-chips should therefore be evaluated by measuring the permeability of the cell barrier. If this permeability is in agreement with physiological levels, then a valuable BBB-on-chip has been obtained which can be used for testing drug candidates. In general, large and hydrophilic molecules, for example dextrans and ions, are physically blocked by the tight junctions between endothelial cells. Ions and essential nutrients such as glucose, amino acids, peptides and hormones are transported actively into the brain by carriers and receptor mediated transport. Small lipophilic molecules (MW < 400-500 Da) can cross the BBB without significant obstruction. However, multidrug resistance transporters regulate efflux of potentially harmful agents, including lipophilic agents. To assess the full barrier function, analytes from all these classes have to be tested in the device: both hydrophilic and lipophilic molecules that are both passively and actively transported or excreted.
Conclusion
The use of BBBs-on-chips has great potential to further the field of BBB research. In microfluidic platforms the advantages of in vivo and in vitro models are combined: organ-on-chip technologies enable the study of organ-level function like in vivo models, while still being robust, reproducible and easy to analyze like in vitro models. There are already a number of reports of BBBs-on-chips in literature that show novel approaches and promising results. These examples already show some benefits of the use of microfluidics for BBB research applications. In addition, organ–on-chip technologies provide flexibility in the design of and control over microenvironments, as well as readout protocols. This enables the development of a wide range of BBB-on-chip models that can each answer specific research questions.